When it is not possible to place the entire component in a furnace for heat treatment (because of the size of the fabrication, circumferential welds in a pipework system or when installing equipment on site, for example), then a local PWHT may be the only option. Local PWHT needs careful planning to ensure that heating and cooling rates are controlled and that an even and correct temperature is achieved. Uneven and/or rapid heating can give rise to harmful temperature gradients producing thermally induced stresses that exceed the yield stress. This may result in the development of new residual stresses when the component is cooled.
Local PWHT may be carried out using high velocity gas burners, infra red burners, induction heating and high or low resistance heating elements. Electrical equipment is more easily installed and controlled than heating using natural gas or propane, particularly on site. High voltage resistance heating is rarely used on site due to the need for the radiant heaters to be positioned a set distance from the surface and, more significantly perhaps, the health and safety risks involved with the use of high voltage current. Low voltage electrical resistance heating and induction heating are the two most commonly used methods.
High velocity gas burners are more advantageous when large areas need to be heat treated, particularly if, for example, firing can take place within a pressure vessel which then becomes its own furnace. For local PWHT of vessel circumferential seams internal insulating barriers can be used to localise the heat source. Motorised valves and micro-processor control of the combustion conditions enabled precise management of the heating cycle to be achieved.
Low voltage electrical resistance heating uses flexible ceramic heating elements, colloquially known as corsets, an appropriate number being assembled to cover the area to be heat treated. Induction heating uses insulated cables that can be wrapped around the joint or shaped to fit the area to be heated or specially designed fitting for repetitive PWHT operations as illustrated in Fig 1.
To perform the PWHT, temperature control thermocouples are firstly attached, often by capacitor discharge welding, the elements placed in position and the area then lagged with thermal insulating blankets to reduce heat loss and to maintain an acceptable temperature gradient.
There are no standard terms used to describe the various regions within the locally PWHT’d area. In this article the terms ‘soak band’, ‘heated band’, ‘gradient control band’, ‘temperature gradient’, which may be axial and through thickness, and ‘control zone’ as suggested by the ASME will be used (see Fig 2).
The soak band is the area that is heated to, within the specified PWHT temperature and time range. It comprises the weld, the two HAZs and part of the surrounding parent metal. The heated band is the area covered by the heating elements, the temperature at the edge of the heated band generally being required to be at least half that of the soak temperature.
The temperature gradient control band is the region where thermal insulation, perhaps supplemented by additional heating elements, is applied to ensure that an acceptable axial temperature gradient is achieved from PWHT temperature to ambient.
A control zone is the region where a number of heating elements are grouped together and controlled by a single thermocouple, enabling different regions to be heated independently; particularly useful with large diameter items or where there are variations in thickness.
Temperature gradients may be axial (along the length of a pipe or vessel) and through thickness. The through thickness temperature gradient is caused by heat losses from the internal surface and is a function of both thickness and internal diameter, the larger the diameter, the greater the effect of radiation and convection losses. Both the width of the soak band and the temperature achieved can be substantially less than that on the outside of the pipe or tube. Insulation on the inner surface will reduce the temperature/width differential but may not be possible on small diameter tubes or pipework systems.
This through thickness gradient is one of the reasons that specifications and codes require the soak or heated band to be a minimum width, generally related in some way to the thickness of the component.
As mentioned above, there are rules in the application codes concerning the size of the heated area, normally related to the thickness. In a circular component such as a pipe butt weld or a pressure vessel circumferential seam the width of the band is easy to calculate. ASME VIII for instance requires the soak band width to be twice the thickness of the weld or 50.8mm either side of the weld, whichever is the lesser.
ASME B31.3 requires the soak band width to be the weld width plus 25.4mm either side of the weld. BS EN 13445 does not specify a soak band width but instead specifies a heated band width of 5√Rt centred on the weld and where R = component inside radius and t = component thickness. There are no requirements in the ASME codes regarding heated band width. A very approximate rule of thumb for flat plate is that the heated band should be a minimum of twice the length of the weld although practical considerations may prevent achieving this ideal.
There are no requirements, in any code or specification, on the width of the thermally insulated band although BS EN 13445 recommends 10√Re. It is essential that the relevant specification is referred to for specific guidance on what is required and it is worth remembering that the specification requirements on soak or heated band widths are minimal and very little is lost by ensuring the specified dimensions are comfortably exceeded.
What is an acceptable axial temperature gradient? Again, there is little advice in the codes and specifications. It is generally assumed that if the temperature at the edge of the heated band is above half that of the soak temperature then the temperature gradient will not be harmful. During heating and cooling BS EN 13445 specifies a maximum temperature difference of 150°C in 4500mm below 450°C (1°C in 3mm) and 1000C in 4500mm above 4500C (1°C in 4.5mm).
To ensure that gradients and temperatures are controlled within acceptable limits sufficient thermocouples need to be attached to provide both temperature control and recording. For small diameter tubes, eg less than 100mm diameter, one control zone and one recording thermocouple are regarded as sufficient; between 100-200mm one control zone and one recording thermocouple at each of the 12 o’clock and 6 o’clock positions; above 250mm diameter one control zone and one recording thermocouple at each 900 quadrant, 12, 3, 6 and 9 o’clock, are suggested.
These thermocouples should be placed on the centre line of the weld. Thermocouples will also be needed at the edge of the soak band and the edge of the heated band. Ideally, thermocouples should also be placed on the opposite surface to the heating elements to ensure that the correct through thickness temperature has been achieved although this is rarely possible on pipe systems.
It is advisable to double up on the thermocouples to cope with the possibility of a thermocouple failure.
Thermocouples use a hot and a cold junction to measure the temperature, the hot junction being attached to the component, the cold junction within the temperature recorder. For accurate temperature measurement the hot junction must obviously be at the temperature of the component. Errors can be introduced if the junction is not firmly attached, either by capacitor discharge (CD) welding, by mechanically fixing the wires to the component or by overheating of the thermocouple junction.
CD welding of the thermocouple wires gives the most accurate results, particularly if the two wires are separated by 3-4mm. Mechanically attached wires will probably need to be insulated by covering the junction with heat resistant putty to prevent overheating of the thermocouple by the overlying heater. If the wire covering is stripped back then the bare wires also need to be insulated.
It is advisable to specify the positions of the thermocouples on a drawing and to include these within a formal written heat treatment procedure document that covers both the specification and best practice requirements.
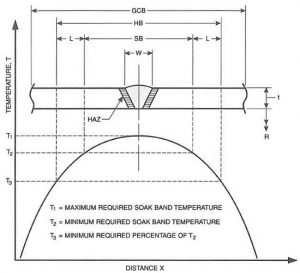
Fig 2 Schematic of Temperature bands within a local PWHT (Reproduced with permission of the American Welding Society (AWS), Miami, Florida, USA)
Stress Relief
Why is it necessary to perform stress relief? It is an expensive operation requiring part or all of the welded item to be heated to a high temperature and it may cause undesirable metallurgical changes in some alloys.
As mentioned above there may be one or more reasons. The high residual stresses locked into a welded joint may cause deformation outside acceptable dimensions to occur when the item is machined or when it enters service.
High residual stresses in carbon and low alloy steels can increase the risk of brittle fracture by providing a driving force for crack propagation. Residual stresses will cause stress corrosion cracking to occur in the correct environment eg carbon and low alloy steels in caustic service or stainless steel exposed to chlorides. What causes these high residual stresses? Welding involves the deposition of molten metal between two essentially cold parent metal faces. As the joint cools the weld metal contracts but is restrained by the cold metal on either side; the residual stress in the joint therefore increases as the temperature falls. When the stress has reached a sufficiently high value (the yield point or proof strength at that temperature) the metal plastically deforms by means of a creep mechanism so that the stress in the joint matches the yield strength. As the temperature continues to fall the yield strength increases, impeding deformation, so that at ambient temperature the residual stress is often equal to the proof strength (Fig 1).
To reduce this high level of residual stress, the component is reheated to a sufficiently high temperature. As the temperature is increased the proof strength falls, allowing deformation to occur and residual stress to decrease until an acceptable level is reached. The component would be held at this temperature (soaked) for a period of time until a stable condition is reached and then cooled back to room temperature. The residual stress remaining in the joint is equal to the proof strength at the soak temperature. Figure 1 shows that residual stress in a carbon manganese steel falls reasonably steadily from ambient to around 600⁰C but that the high strength creep resistant steels need to be above 400⁰C before the residual stress begins to fall. Stainless steel is hardly affected until the temperature exceeds 500⁰C. There is therefore a range of soak temperatures for the various alloys to achieve an acceptable reduction in residual stress without adversely affecting the mechanical properties of the joint.
In carbon manganese steels this temperature will be between 550-620 degree C, in creep resistant steels somewhere between 650-750⁰C and for stainless steels between 800-850⁰C.